Meet our Team: University of São Paulo
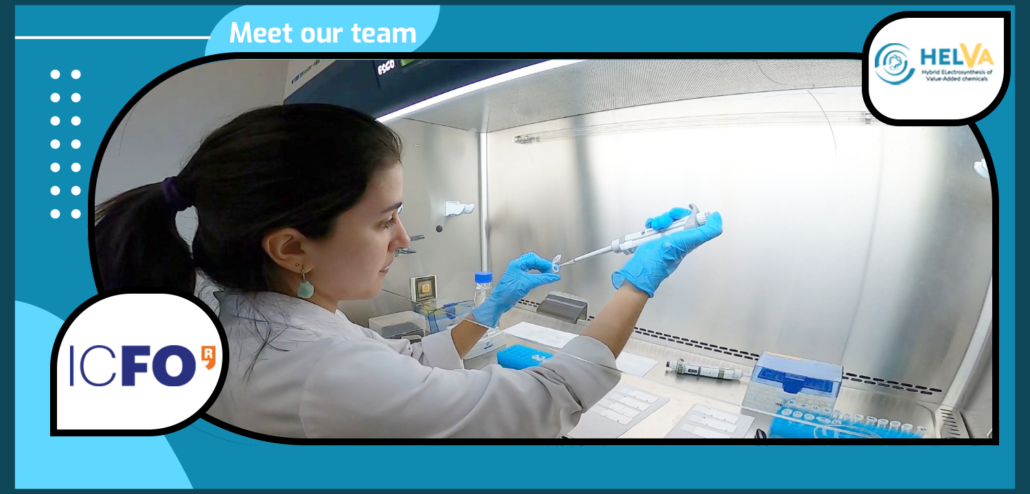
The Fungal Bioluminescence Laboratory (LBF) from the University of São Paulo focuses on studying bioluminescent systems, environmental metagenomics, and introducing useful genes into microorganisms to make them perform specific tasks. In the HELVA project, the LBF team is responsible for isolating and engineering certain bacteria strains capable of producing polyhydroxyalkanoates (PHA), biodegradable polymers that have the potential to replace petrochemical plastics. Their approach combines field sampling of bacterial strains from ecosystems like the Atlantic Forest with laboratory-based genetic engineering to enhance microbial PHA production. For the first chapter of the “Meet Our Team” series, we have interviewed Prof. Cassius Vinicius Stevani, leader of the LBF team, to learn more about their research and expectations and contributions to the HELVA project.
Prof. Stevani, could you first briefly introduce your research group and its primary areas of expertise?
Our research group at the Fungal Bioluminescence Laboratory (LBF), located in the Institute of Chemistry at the University of São Paulo, focuses on three main areas: mechanistic and applied studies on bioluminescent systems, particularly those of fungi; environmental metagenomics; and heterologous expression of biotechnologically relevant genes in microorganisms. Our team includes collaborators, students, and postdoctoral researchers with diverse backgrounds, spanning organic chemistry, microbiology, photochemistry, and molecular biology. Our work includes both field and laboratory experiments. We collect samples from the Atlantic Forest, one of Brazil’s biodiversity hotspots, from caves, ponds, and water reservoirs.
Which is your specific role and contribution to the project?
Our role in the HELVA project involves prospecting for and engineering bacteria to produce polyhydroxyalkanoate (PHA), a biodegradable polymer. We search for PHA-producing bacteria during our fieldwork, followed by isolation and identification of those with this production capability. PHA production can also be enhanced through genetic manipulation and optimization of cultivation conditions.

What are the primary challenges your group is facing in achieving its objectives within the first year of HELVA?
Our primary challenges involve prospecting and isolating bacterial strains capable of producing PHA from natural environments, such as the Atlantic Forest, and optimizing culture conditions to enhance PHA production. Additionally, adapting these strains, along with engineered strains like E. coli, to efficiently utilize acetate as a substrate presents both technical and biological challenges, particularly in scaling these processes for consistent results.
How are you overcoming these challenges or mitigating their impact?
We are addressing these challenges through systematic fieldwork and screening efforts, focusing on diverse environments to capture a wide array of bacterial candidates. In the lab, we’re employing iterative optimization of culture media and conditions to improve PHA yields. Genetic engineering techniques are also being used to enhance strains like E. coli, which allows us to increase PHA production through targeted modifications, while testing these strains under various acetate concentrations to improve their adaptability and productivity.
What are the main achievements so far?
Our team has successfully isolated two promising bacterial strains from Cupriavidus and Pseudomonas genus with potential for high-yield PHA production. We’ve also made significant strides in optimizing culture conditions to promote PHA accumulation using acetate as a primary carbon source. In parallel, preliminary genetic modifications in E. coli have shown promising results in increasing PHA yields, bringing us closer to scalable production.

What are the key techniques your group is employing in the project?
Our approach relies heavily on microbial isolation techniques, followed by molecular identification methods, such as 16S rRNA sequencing, to classify potential PHA-producing bacteria. For optimization, we use culture testing under variable conditions, while advanced genetic engineering techniques enable us to modify metabolic pathways in E. coli and other strains to increase PHA production. Analytical techniques, like gas chromatography and mass spectrometry, are also integral for monitoring and quantifying PHA output.
How does your group’s expertise can contribute to the overall success of the HELVA?
Our team’s expertise in microbial prospecting, molecular biology, and metabolic engineering uniquely positions us to contribute to HELVA’s goals. By isolating new, naturally occurring PHA-producing bacteria and enhancing production pathways in model strains, we provide foundational data and biological resources critical for developing scalable, sustainable bioplastic production.

Why do you think it is important for your research team to take part in this project?
This project aligns closely with our commitment to leveraging biodiversity for sustainable innovation. By participating in HELVA, we help develop solutions rooted in the natural resilience and adaptability of microbial systems, adding value to sustainable material research and highlighting Brazil’s unique ecosystems as sources of novel biotechnological applications.
What is the most interesting part of the work you are doing within HELVA?
The exploration of microbial diversity in the Atlantic Forest and other ecosystems, combined with the challenge of optimizing and engineering these strains for PHA production, is exceptionally intriguing. It is a unique opportunity to bridge natural biodiversity with engineered biological systems, creating a path toward sustainable material production through bioplastics.
Finally, what are your expectations for the HELVA project in terms of its long-term impact on the field of sustainable materials?
We anticipate that HELVA will drive significant advancements in sustainable materials by introducing bioplastics as practical alternatives to petrochemical plastics. In the long term, our work could establish a blueprint for harnessing environmental microbial resources to create bioplastics, promoting sustainability and contributing to global efforts in circular bioeconomy models.